The age of discovery is not over.
Once, scurvy-riddled Europeans sailed into the unknown to claim foreign, fantastic parts of the world.
Now, physicists sit in labs and ask, “Is this all there is?”
No, they aren’t suffering a collective existential crisis.
They’re looking for dark matter—the stuff that theoretically makes up a quarter of our universe.
And West Aussie researchers are at the forefront of this search, as part of an Australian-wide project to detect a particle called the axion.
WHAT’S THE (DARK) MATTER?
If dark matter exists, you are probably sitting in a soup of it right now.
Scientists predict it makes up 26.8% of the universe, which is pretty significant when you consider that everything else we can observe—from hydrogen atoms to black holes—makes up only 5%.
(The other 69% is something scientists call dark energy. Don’t worry about it.)
There’s just one problem.
It doesn’t interact with electromagnetism—the force between positively and negatively charged particles. It’s responsible for practically everything we can observe in day-to-day life—with the exception of gravity.
Electromagnetic forces present between atoms and molecules in the ground is the reason Earth’s gravity doesn’t keep pulling us all the way down to its (molten hot) core.
The light being emitted from your computer, allowing you to read this story, is generated by interactions of electrically charged particles in your monitor, otherwise known as electricity.
Ordinary matter looks like ordinary matter because of the electromagnetic forces between atoms and molecules.
But dark matter doesn’t interact with electromagnetism. That means we can’t see, smell, taste or touch it.
So if dark matter is essentially undetectable, why do we think it exists? And what on Earth are we looking for?
IN THE DARK
Let’s start with a basic assumption—gravity exists.
Along with electromagnetism, gravity is one of the four basic forces that physicists use to explain almost everything. Gravity says that heavy things attract all other heavy things, so Earth’s gravitational pull is the reason we aren’t all floating aimlessly in space.
If we peer into all that space, we can see that our Milky Way galaxy is spiral shaped. Smack bang in the galactic centre is a big, bar-shaped bulge from which spiralling arms snake around in a flat circle. Earth sits somewhere in the middle of one of those arms and completes one lap of the galaxy every 225 to 250 million years.
If we think about the entire universe as a giant amusement park, we can imagine our Milky Way to be a carousel. Unlike normal carousels that have plastic ponies fixed in place by poles, the stars, moons and planets that make up our galaxy are disconnected and free to spin around at different speeds.
So if everything is disjointed and spinning, what’s keeping us orbiting neatly in our little spiral?
Well if we continue with the theme park analogy, we can liken this phenomenon to a swing chair ride.
When swinging in a chair around a tower, a metal chain provides a constant force into the centre of the ride that keeps you spinning round and around that central pole.
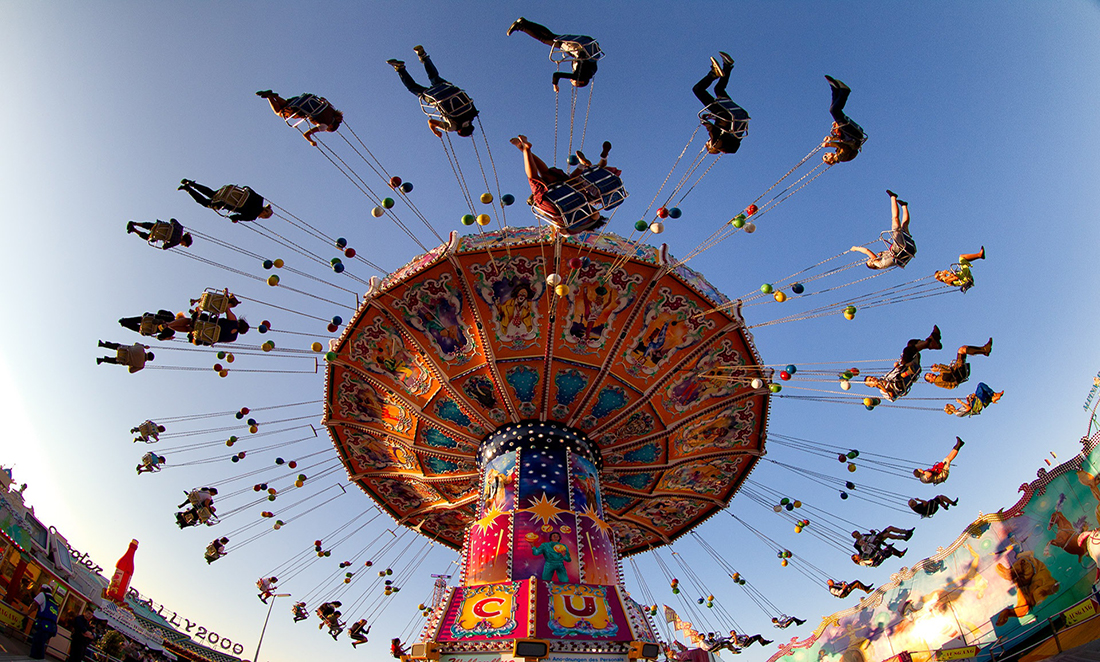
The same sort of thing occurs in space, except instead of a chain, we’ve got gravity. Gravity is provided by the mass of stuff—specifically, the mass of our galactic centre, which scientists believe to be a supermassive black hole. It has so much mass in so little space that it exerts a gravitational force so high it sucks in light.
When you move away from the centre and into the flat galactic halo, we see a lot less stuff. Less stuff means less mass, which means less gravity. We could therefore expect the stuff in the spiral arms to be spinning slower than the stuff closer to the middle.
What astrophysicists actually see is that things on the outer edge of the galaxy are spinning at the same rate as things near the centre of the galaxy—and that’s pretty damn fast.
If this was the case in our theme park, we would have slipped into a nightmare scenario.
The spinning chair ride would be whirling around so fast that the chain would no longer provide enough force to keep you moving in a circle. The chain would break, and you would be flung to a death worthy of a B-grade horror movie.
The fact that Earth has not been slingshotted far and wide suggests that we are surrounded by a lot more mass, which provides a whole bunch of gravity and keeps our galaxy in shape.
And most physicists think that mass might just be dark matter.
DARK CANDIDATES
Just for a second, forget everything you just read.
We’re going to stop staring at stars and instead investigate much smaller things—particles.
Particle physics is home to this problem called the strong charge parity (CP) problem. It’s a very big unexplainable problem in the otherwise successful theory of quantum chromodynamics.
Don’t worry about it.
Using mathematical equations, particle physicists in the 70s suggested we could solve this strong CP problem with the introduction of a theoretical particle called the axion.
And if we do more maths and write a description of what the axion particle should look like, we would find that it has two very exciting qualities—a) it has mass and b) it does not interact with electromagnetism very much at all.
Which sounds suspiciously like the qualities of dark matter.
The axion is what physicists call a ‘promising candidate’ for dark matter. It’s like killing two birds with one theoretical, invisible stone.
And if axions are dark matter, we should be surrounded by them right now.
If we could only build the right equipment, we could perhaps detect the mysterious mass that’s holding our galaxy together.
As it happens, some clever scientists at UWA are doing just that.
DARK MATTER TURNS LIGHT
Physicists at a UWA node of the ARC Centre of Excellence for Engineered Quantum Systems (EQuS) are employing a piece of equipment called a haloscope—so called because it searches for axions in the galactic halo (which you’re sitting in right now).
A haloscope is basically an empty copper can (a ‘resonant cavity’) placed in a very cold, very strong magnetic field.
If axions are dark matter and exist all around us, one might enter the resonant cavity, react with the magnetic field and transform into a particle of light—a photon.
Whilst we wouldn’t be able to see these photons, scientists are pretty good at measuring them. They’re able to measure how much energy it has (its frequency) as it sits inside the resonant cavity. And that frequency corresponds to the mass of the axion that it came from.
The problem is, resonant cavities (those empty copper cans) are created to detect photons with specific frequencies. We don’t know how heavy axions are, so we don’t know what frequency photon they will produce, which means building the right resonator involves a bit of guesswork.
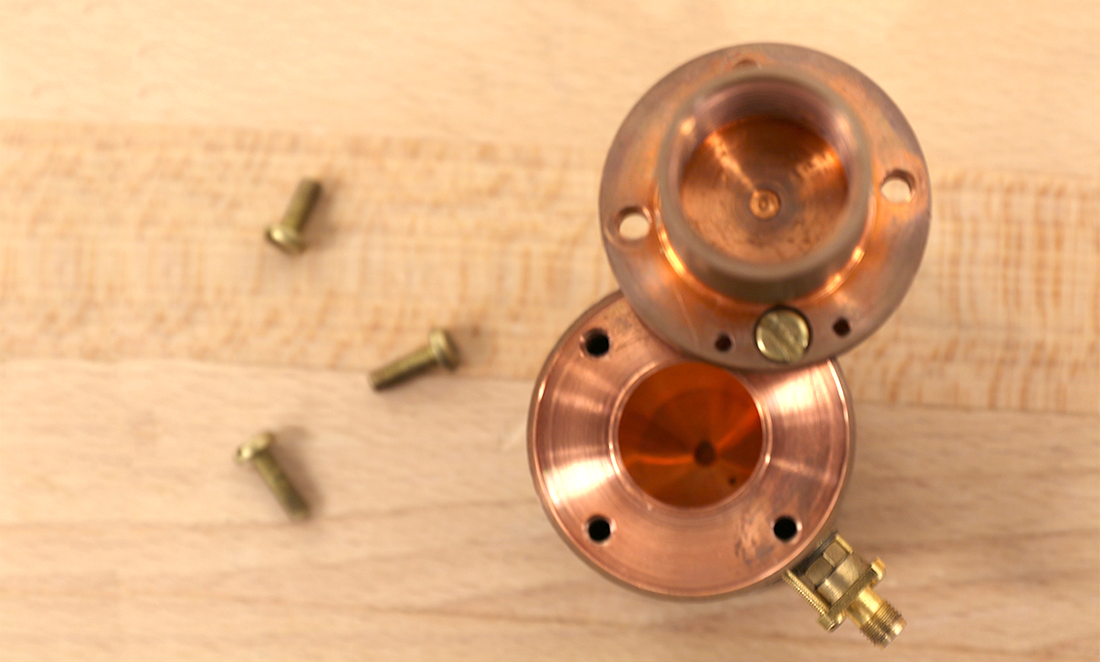
The search for the axion is more of a process of elimination.
What have they been able to exclude so far?
Well (mostly due to technical limitations) scientists have previously been looking for axions with a low mass.
New theoretical models predict that the axion is a bit heavier.
How heavy? We don’t know.
But Aussie researchers have just been awarded 7 years of funding to try and find out.
SCOPING THE HALO
The Oscillating Resonant Group AxioN (ORGAN) experiment is a nationwide collaboration between members of EQuS and is hosted at UWA.
Part of the physicists’ work over the next 7 years will be to design resonant cavities that are capable of detecting heavier axions.
They ran an initial experiment over Christmas 2016, the ORGAN Pathfinder, to confirm that their haloscopes were up to the task ahead and that the physicists were capable of analysing their results.
This experiment yielded no results—but that doesn’t mean that axions don’t exist. It only means that they don’t exist with the specific mass that they searched for in December 2016 and to a certain level of sensitivity.
The intrepid explorers at UWA will set sail into the next stages of the ORGAN experiment in 2018.
And perhaps soon, we’ll know exactly what the matter is.